HISTORY
It is an unbelievable sixty years since the writer, Harold Harris and Tony Slota started Mason Industries with Pat Lama joining ten years later. Unfortunately, both Harold and Tony are gone and Pat retired. So at the young age of ninety, I continue to run the company with Doug Valerio’s help. sixty years of continuity and ownership.
We offer our thanks to every contractor and manufacturer who forms our customer base. We believe they favor us because of the practicality of our designs and lower installed cost when product works the first time. We stand behind what we sell as no other competitor. We thank our whole representative organization for their hard work, professionalism, integrity and loyalty. We look forward to serving you.
1945
It has been my pleasure to serve on vessels driven by 3 cylinder reciprocating steam engines, which powered our Liberty Ships just as they did in World War I to Twin Diesel Nordberg engines, steam turbines and perhaps most interesting of all, an opposed piston diesel engine with 36 inch diameter pistons and a 6 foot stroke that ran at the ship’s screw speed of 92 RPM. It was so large you could walk into the crank case to check the bearings and cross heads. As ship’s machinist, I worked with lathes, milling machines, drill presses, shapers and hydraulic presses as well.
After discharge, I returned to CCNY, got my degree in Mechanical Engineering and five years later, my license as a New York P.E.
1948 was a terrible year for engineers, and jobs were hard to come by. One thing I knew was that I did not want to become a “salesman,” and needed to find something that was hands-on rather than only design.
I was more fortunate than most, because I could continue to work as a night engineer on ships in port. This meant you could sleep, but were on call in an emergency. I earned $85 a week for sleeping while hunting for a $26 job, which is what engineers started at in 1948.
When this went on for six weeks or so, my father suggested I put an ad in the “Positions Wanted” column instead of continuing to depend on the Employment Agencies and answering advertisements. I told him it was useless as all potential employers were only interested in five years experience. He did not agree as he thought the practical marine experience was priceless and my enthusiasm to take on physical work unusual. So as in normal father son relationships, he placed the advertisement on his own. Mark Twain said something like “When he was sixteen, he couldn’t imagine how simple his father was and survived. Then he was amazed by the time he was thirty at how much his father had learned.”
We received over twenty responses and one of them was the sales agency for the Korfund Company, so far as I know, the largest and oldest vibration isolation company in the country in 1948. The name Korfund was the German abbreviation for “Cork Foundation.” Cork pads were still very acceptable, so the name fit.
Without the slightest idea what “vibration isolation” was, I weathered the interview. Since it was all meaningless, I asked what kind of position they were trying to fill. The answer was “Vibration Control Sales Engineer.” So cocky twenty-four year old devil that I was, I stood up and started to leave. I knew I did not want to be a Sales Engineer.
Fortunately, one of the two partners was a very bright sarcastic person. He said he realized I was extremely busy with hundreds of interviews lined up, but could I possibly spare him another ten minutes, so I apologized and sat down. He reversed the question and asked what it was I would like to do.
I told him that I thought I would like to work for a contracting office where part of the time would be designing and if the rest were hitting a star drill with a three pound sledge, that would really be a winner. With that,
his face lit up and he said when they explained the physical aspect of their business, everyone else had walked out.
It turned out since so little isolation was used in those days, the only way they could be successful was to become isolation contractors. Instead of selling a set of mountings, they would analyze the machine that was causing difficulty and quote the isolation installed and guaranteed to solve the problem or not get paid. In addition to being salesmen, they had trained themselves to be damn good riggers and I jumped at the opportunity.
While Korfund was the best at the time, many of the mountings and hangers did not have deflections of more than 5/8” and most springs were in housings that bound and would not do the job. I had the engineering passion to solve problems and my employer the financial interest of not walking away when standard product did not work. Never caring about hours, I started to design at night using new concepts. Korfund would then manufacture to our drawings.
Since we were only the New York agents, it got to be the tail wagging the dog, and there was no choice but to leave and start Vibration Mountings, Inc. where I was a Junior Partner. VMC is still in business today, and many of their existing designs reflect my work up until 1958. While I was a Junior Partner, there was a basic conflict of interest. My belief had always been that if you manufacture a superior product, the market will recognize and buy it. This has never changed and the constant resistance by my Senior Partner, who was only interested in “the bottom line,” led to my becoming ill from frustration. The only way to solve that was to set up Mason Industries after ten years of installation and design experience.
1968 - ASHRAE LECTURE
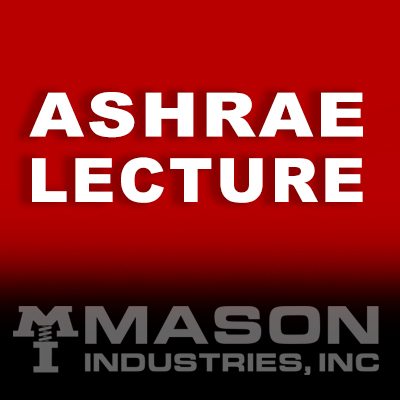
Until a very few years ago the vibration isolator recommendations for air conditioning equipment were more a matter of tradition than science. It was well established in the industry that cork was best under high speed equipment such as pumps and centrifugal compressors, rubber mountings ideal for fans and air handling equipment, and spring mountings the logical choice for reciprocating equipment such as compressors. These selections were so well established that the application engineer found his decisions concerned a choice of materials within the categories, such as double rather than single deflection rubber mountings. Cork materials were manufactured and sold in natural form and also in precompressed sheets. The natural form of cork was white and the precompressed sheets were brown. One material really worked no better than the other but a decision had to be reached on which color to use. For all the difference it made, decorator colors should have controlled.
This medieval method of engineering served its purpose as it introduced the vibration control industry to this country. Oddly enough installations were successful, at least financially, as long as the equipment was installed in the basement or on grade; as long as the HP involved was small; as long as the public was not too demanding, and as long as building structures followed the pattern established in the early nineteen hundreds when floors were thick, spans were short and the curtain wall probably didn’t exist.
Sometime following the World War II years, a general conspiracy developed to embarrass the vibration control people. The air conditioning industry really came into its own, and entire buildings were air conditioned rather than just limited spaces. Basement space, previously considered a machinery or storage area, became rentable for offices or parking. This meant that the air conditioning machinery had to be moved somewhere in the building, and more often than not, it ended up in the penthouse under the cooling towers and over executive areas. In many cases, air distribution components such as high pressure fans or high pressure cabinets were located immediately adjacent to office space on the intermediate floors and the vibration problem was exaggerated by the accompanying change in structural design. Buildings were made very much lighter and the floor spans were increased to provide unobstructed space for offices. The old traditional method of mounting selection fell down on job after job and bad installations plagued the vibration control industry.
All of this ushered in a theoretical design era based on an overly simplified vibration control equation. This equation as discussed below merely says that a given percentage of the vibration can be kept out of the structure by installing the machine which is creating a vibration at a known frequency (usually the rpm of the machine) on a system of vibration mountings that want to resonate at a frequency which is very much lower than the disturbing frequency as described above. When this ratio of the disturbing frequency to the natural frequency is about 3 to 1, 90% of the vibration is eliminated theoretically.

All of the vibration companies published an Efficiency Chart as shown in Figure 1, page 3, which expressed this relationship. Since the disturbing frequency was always known and unvariable, one merely had to establish the natural or resonant frequency by proper mounting selection. Fortunately, just as a pendulum’s frequency is a function of its length rather than the weight of the bob at the end, an isolation system’s frequency is a function of the static deflection in the supporting medium, rather than the weight of the system. In order to provide 90% isolation efficiency for a compressor running at 600 rpm, you merely selected a mounting that had about an inch of deflection which would result in a base frequency of 188 cycles per minute. The ratio of about 3 to 1 was established and the problem was solved. The vibration companies then advocated specifications which would read “Vibration mountings shall be selected to eliminate 90% of the vibration transmitted by the air conditioning equipment.”

Schematic of the Single Mass System Referred to in the Theoretical Efficiency Chart

While this approach was a tremendous leap from the nothing era, it proved to be only a starting point for a number of reasons. First, the 90% requirement gave no consideration to the magnitude of the vibration. In approaching any problem we must realize that the vibratory force varies directly as the unbalanced weight, directly as the distance the unbalance is located from center and as the square of the velocity or the rpm. The vibratory force created by an 18″ vent set running at 300 rpm. is nothing as compared to the vibratory force from a 60″ class-3 fan running at 1000 rpm. While a 90% efficiency might be an exaggerated number for the vent set, it might very well be completely inadequate for the large high speed fan. But the grossest error appeared because the selections were made on the false premise that the theoretical equation applied to structures as we know them today. Unfortunately this is not true, as the equation is based on the assumption that the deflection in the vibration isolator is extremely large as compared to the floor deflection, and that the moving mass of isolated equipment is extremely small as compared to the floor mass. While these assumptions were closer to being correct in the old buildings with smaller equipment, they are seldom valid today. We are dealing with 2-mass rather than single-mass systems. The floor has a mass and a spring rate of its own as schematically illustrated in Figure 2. This system cannot be analyzed in terms of the simplified theoretical equation. In using the theoretical equation, in many cases you will select a mounting which

has a smaller deflection than the floor system on which it is sitting. For example, if we decide to isolate a 3600 rpm. pump to eliminate 95% of the vibration, we would pick mountings with deflections of about 0.05″. Floors are designed with an allowable deflection which is normally as much as 1/360 of the free span. Twenty foot bays are small today, but assuming this pump were located in a 20′ bay, the floor deflection might be as much as 1/360 x 240″ or approximately 0.666″. If the floor had only half the maximum allowable deflection, it would still be 6.5 times less stiff than the isolator we have selected. The introduction of this stiff mounting will do nothing for the floor isolation.
There are more complicated equations that can be used to theoretically solve any vibration application problem. This type of equation is useful occasionally to find out if we are working in the proper direction when a new system of isolation is developed. This type of analysis has shown that to get back to the theoretical figures shown by the Efficiency Chart, the isolator deflection has to be as much as 6 – 8 times the floor deflection when the slab is light. While these numbers may sound far fetched, in actual solutions of existing problems, we have used mountings with as much as 3″ of static deflection to solve a problem where the theoretical charts showed us that a 3/8″ deflection should have been quite satisfactory.
Today’s deflections are selected on an empirical basis based on actual field experience. These are practical values that are necessarily broad in scope. In some cases they may be more than is actually required to solve a particular problem, but a commercial vendor must deal in broad terms. On large critical jobs it is often important to rely on the impartial professional judgment of a qualified acoustical consultant to make a specific job analysis and sort out the exact requirements for each piece of equipment depending on its size and location in a particular type of structure. The commercial vendor is dedicated to his own company and usually suffers from limited acceptance of his competitor’s best offerings. The professional is free of this prejudice and can make his selections from designs submitted by the entire industry. A consensus of the deflections we have worked to for various floor spans are shown in the Selection Guide at the end of the Engineering Specification Bulletin.
This need for higher deflection materials has caused reclassification of products: Pad materials, be they neoprene, cork, combinations of cork and neoprene, fiberglas, sisal fibers, felt, lead or any other material, provide limited deflections. These deflections are normally 10-20% of the pad’s thickness. Therefore, pads are good for high frequency noise breaks, and since their deflections are almost always small in comparison to upper floor deflections, their use should generally be confined to basement areas, non-critical jobs or situations where job costs must be kept to an absolute minimum regardless of performance.
Neoprene mountings and hangers fall into the 0.20″ to 0.50″ deflection range. They do provide sufficient static deflection to offer protection under small high-speed equipment such as close-coupled pumps up to 3 HP, vent sets, small heating ventilating units, etc., where the unbalanced forces are so small that in all probability only a noise break and minor vibration relief need be provided. Neoprene hangers are sufficiently effective for isolation of steam lines where there is seldom any real vibration, but only high frequency whistles and noises of that nature. While neoprene mountings and hangers do have their place as noted, there is a tendency for specifications to call for spring mountings throughout because the dollar difference between the neoprene mountings and small spring mountings is a very small percentage of the overall job cost. Neoprene hanger elements are often used in series with springs, because the neoprene element will do a far more efficient job of eliminating the high frequency noises than the spring alone.
Steel spring mountings are by far the most widely used commodity on critical jobs today. Steel springs are practical through 5″ of static deflection and even more on specific occasions. Springs provide an easily variable design medium, and steel spring installations are as permanent as the machine itself when selections are made within proper stress values. Most modern isolators are simply steel springs that have been designed with large enough diameters to provide stability without the need for a supplementary, often detrimental, housing. They are generally manufactured with an adjustment bolt and a pad made of neoprene or some other material in series with the spring to attenuate the high frequencies.
A relative newcomer to the field of air conditioning equipment isolation is the Air Spring. In broad terms an Air Spring is merely a large bladder that is manufactured in a configuration to withstand as much as 100# of air pressure and provide a stable support point for the equipment. By properly shaping the air spring, small units can be designed to provide the equivalent of 6” to 7” of spring deflection in the steel spring series. Since the walls of the air spring are constructed of rubber, there is no possibility of the type of spring resonance sometimes found in the coils of large deflection, large diameter steel springs. Air springs have the advantage of supporting a wide range of loads merely by varying air pressure, and the spring frequency is a function of the shape rather than the pressure. In general, air springs are installed with a replenishing air supply to compensate for leakage, or expansion and contraction where there is a wide temperature variation. Height Control valves are provided to maintain elevation and compensate for external forces such as fan thrusts. Since these complete installations are generally more expensive than steel spring isolation methods, air springs are generally reserved for extremely critical locations as recommended by an acoustical consultant.
Supplementary steel or concrete equipment bases are most often used to keep equipment in alignment. This may be true when the base is used to tie a fan and motor together, or provide the common base for a turbine-driven compressor. Bases may also be used to stiffen an existing base such as a cast iron pump base, provide stability for tall machines such as absorption machines, or tie a complete package together as in the case of a long, many-sectioned heating and ventilating unit. In many cases, bases are constructed of steel rather than concrete because they can be shipped to the job as a complete welded assembly and installed without involving more than one trade. Their light weight as compared to concrete, lessens the floor burden and reduces the need for strengthening the floor slab. When steel bases are used, however, it is most important that they are made sufficiently rigid to provide support for the mechanical equipment and do not resonate at the frequency of the equipment they are supporting. The best approach to this is the use of steel beams as the base members, and we would suggest a depth of framing equal to 1/10 the longest dimension of the base. Cross-framing may be used for additional stiffening especially on large HP direct-drive units.
Floating concrete bases are recommended for pumps because pump bases are designed for grouting to concrete floors. The use of a floating concrete base provides a grouting surface and the extra stiffness the pump base requires. When stiffness is the only factor, a concrete depth equal to 1/12 the longest dimension of the base is usually satisfactory. Concrete bases are also used for inertial purposes when an increase in mass is necessary to resist either the unbalance of the equipment or external forces. The classic example of unbalanced equipment is the single or double cylindered vertical or horizontal slow-speed air compressor. These large bore, long-stroke machines usually run at 350 rpm and they can be only partially balanced. The weight of the concrete base is calculated from the unbalanced force information supplied by the compressor manufacturer. These concrete inertia bases are 5 to 7 times the weight of the compressor in order to bring the motion down to acceptable limits.
Concrete bases can be used to offer resistance to external forces such as fan thrust. We have found that this is particularly important when isolating high pressure cabinet units because there is a combination of back vacuum and fan thrust which tend to displace them. Concrete bases are suggested for the isolation of high pressure fans above 6“static pressure. The weight requirement falls somewhere between 1 to 3 times the weight of the equipment and must be determined in each situation.
While simple steel bases are generally more economical than concrete filled bases, most of our specifications call for concrete as a more foolproof broad application method.
Flexible hose should be installed horizontal and parallel to the axis of rotating equipment. This allows the hose to flex in the transverse direction. All hoses are quite stiff axially. Butyl rubber hoses have a better chance of reducing pipe wall transmitted noise, but we have found no hose particularly effective against water-borne disturbances. When butyl hoses are used, we would suggest that Control Cables are installed parallel to the hose to prevent elongation and possible hose failure. Stainless steel or bronze metallic hoses are recommended for those services where the static pressure or temperature exceeds the capability of the rubber materials. While flexible hoses are not complete protection against pipe line noises, they do provide flexibility at the points of connection to the mechanical equipment. This reduces stresses to the flanges and allows the isolated equipment to move freely on the springs.
Since noises transmitted to pipe lines will travel for unpredictable distances, the trend is to isolate mechanical pipe lines throughout their run. This type of isolation is normally provided by combination spring and neoprene hangers.
While all connections to high pressure ducts should be made flexible, these connections are not completely successful either. There is enough air turbulence in high pressure systems to vibrate the ducts and cause possible transmission to the structure. Ducts are normally isolated by 1″ deflection spring hangers that are installed up to 50′ from the fan discharge.
The following specifications describe commodities that are now common to the industry. They are available from many of the manufacturers. Our selection guide refers to these commodities with suggested minimum static deflections as noted. This guide is provided as a broad introduction to the field of specifications and we recommend its use to develop a general feel for the subject and application on projects of an everyday nature. We hope that your being familiar with these designs and recommendations will help you in your work and that we can continue to contribute to the general pool of vibration control information.
1971
When we opened our doors in 1958, we rented 3,000 square feet. Our catalog was a black pasteboard cover enclosing six pages and held together with brass fasteners. However, we had copied nothing and this hands-on originality and enthusiasm was greeted with open arms. In a short time we built platforms over the whole shop to gain more production space. Since our foreman could not pronounce “mezzanine,” they were referred to as “mezzerines” from then on. We broke through the factory wall and gained another adjacent 4,000 square feet, and soon filled that with “mezzerines” as well. (Incidentally, our original capitalization was $24,000. So how we managed is a story unto itself.)
Around 1968 we pulled wagons down the street and moved ourselves into a 30,000 square foot building and sure enough that was soon covered with “mezzerines” too. We then broke through the walls again and displaced an adjacent body repair shop until we ran out of “mezzerines.”
1981 - New Office in NY
In 1981 we bought and moved into our present 60,000 square foot headquarters, and yes, it is now full of “mezzerines.” We rent an additional 50,000 square feet in two buildings nearby to house our tremendous inventory and there is an additional 150,000 square feet Overseas.
The New York and Los Angeles offices and Engineering staff number approximately eighty people of which twenty-one are engineers, about half are licensed. With all production workers included, we employ approximately three hundred people.
We are more than capably represented by about eighty firms within the United States and around the world.
2004
Up until the age of sixty-five I was still installing product, so virtually every design is still a problem solver with many at the request of seismic or acoustical engineers to fill their needs in a new area.
Never for a moment do I believe that we could have done this without the tremendous support provided by the professional community that consist of thousands of HVAC engineering and architectural firms as well as acoustical consultants. We have addressed hundreds of ASHRAE Chapters and many national meetings. Without their recognition of our constant effort to improve the technology and suggested specifications, we would still be in that 3,000 square foot 1958 space, and probably without the “mezzerines.”
We also offer our thanks to every contractor and manufacturer who form our customer base. We believe they favor us because of the practicality of our designs and lower installed cost when product works the first time. We stand behind what we sell as no other competitor. We thank our whole representative organization for their hard work, professionalism, integrity and loyalty.
2006
A vertical loop has always been a very useful and popular hose configuration and is still used in small diameters. If the radius is generous and considerably larger than the minimum bend radius. (i.e.: Hose forced into an arc less than the minimum bend radius takes a permanent set.) The configuration can move in many interesting ways as sketched.
The degree of motion is dependent on the diameter, length of live hose and theradius. Hoses that are more flexible because of more corrugations per foot, will accept greater displacements for a given configuration.
It is not practical in the larger diameters as the bend radii become large and the configuration takes too much space, because of what is lost to the semicircle at the bottom. Typical bend radii are as follows for intermittent flexing. A fixed bend could be tighter.
As a 6” diameter hose would have a 56” diameter semicircle at the bottom, plus the vertical legs, you can see the problem.